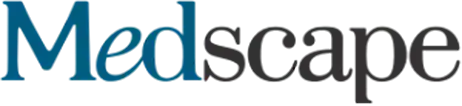
Drugs & Diseases > Hematology
Iron Deficiency Anemia
Updated: Nov 14, 2016
Author: James L Harper, MD; Chief Editor: Emmanuel C Besa, MD more...
Share Feedback
SECTIONS
Practice Essentials
Iron deficiency anemia develops when body stores of iron drop too low to support normal red blood cell (RBC) production. Inadequate dietary iron, impaired iron absorption, bleeding, or loss of body iron in the urine may be the cause. [1] Iron equilibrium in the body normally is regulated carefully to ensure that sufficient iron is absorbed in order to compensate for body losses of iron (see the image below).
The total body iron in a 70-kg man is about 4 g. This is maintained by a balance between absorption and body losses. Although the body only absorbs 1 mg daily to maintain equilibrium, the internal requirement for iron is greater (20-25 mg). An erythrocyte has a lifespan of 120 days so that 0.8% of red blood cells are destroyed and replaced each day. A man with 5 L of blood volume has 2.5 g of iron incorporated into the hemoglobin, with a daily turnover of 20 mg for hemoglobin synthesis and degradation and another 5 mg for other requirements. Most of this iron passes through the plasma for reutilization. Iron in excess of these requirements is deposited in body stores as ferritin or hemosiderin.
Signs and symptoms
Patients with iron deficiency anemia may report the following:
Fatigue and diminished capability to perform hard labor
Leg cramps on climbing stairs
Craving ice (in some cases, cold celery or other cold vegetables) to suck or chew
Poor scholastic performance
Cold intolerance
Reduced resistance to infection
Altered behavior (eg, attention deficit disorder)
Dysphagia with solid foods (from esophageal webbing)
Worsened symptoms of comorbid cardiac or pulmonary disease
Findings on physical examination may include the following:
Impaired growth in infants
Pallor of the mucous membranes (a nonspecific finding)
Spoon-shaped nails (koilonychia)
A glossy tongue, with atrophy of the lingual papillae
Fissures at the corners of the mouth (angular stomatitis)
Splenomegaly (in severe, persistent, untreated cases)
Pseudotumor cerebri (a rare finding in severe cases)
.
Diagnosis
Useful tests include the following:
Complete blood count
Peripheral blood smear
Serum iron, total iron-binding capacity (TIBC), and serum ferritin
Evaluation for hemosiderinuria, hemoglobinuria, and pulmonary hemosiderosis
Hemoglobin electrophoresis and measurement of hemoglobin A 2 and fetal hemoglobin
Reticulocyte hemoglobin content
Tests useful for establishing the etiology of iron deficiency anemia and excluding or establishing a diagnosis of another microcytic anemia include the following:
Stool testing
Incubated osmotic fragility testing
Measurement of lead in tissue
Bone marrow aspiration
CBC results in iron deficiency anemia include the following:
Low mean corpuscular volume (MCV)
Low mean corpuscular hemoglobin concentration (MCHC)
Elevated platelet count (>450,000/µL) in many cases
Normal or elevated white blood cell count
Peripheral smear results in iron deficiency anemia are as follows:
RBCs are microcytic and hypochromic in chronic cases
Platelets usually are increased
In contrast to thalassemia, target cells are usually not present, and anisocytosis and poikilocytosis are not marked
In contrast to hemoglobin C disorders, intraerythrocytic crystals are not seen
Results of iron studies are as follows:
Low serum iron and ferritin levels with an elevated TIBC are diagnostic of iron deficiency
A normal serum ferritin can be seen in patients who are deficient in iron and have coexistent diseases (eg, hepatitis or anemia of chronic disorders)
Management
Treatment of iron deficiency anemia consists of correcting the underlying etiology and replenishing iron stores. Iron therapy is as follows:
Oral ferrous iron salts are the most economical and effective form
Ferrous sulfate is the most commonly used iron salt
Better absorption and lower morbidity have been claimed for other iron salts
Toxicity is generally proportional to the amount of iron available for absorption
Reserve parenteral iron for patients who are either unable to absorb oral iron or who have increasing anemia despite adequate doses of oral iron
Reserve transfusion of packed RBCs for patients who are experiencing significant acute bleeding or are in danger of hypoxia and/or coronary insufficiency
Background
Iron deficiency is defined as a decreased total iron body content. Iron deficiency anemia occurs when iron deficiency is severe enough to diminish erythropoiesis and cause the development of anemia. Iron deficiency is the most prevalent single deficiency state on a worldwide basis. It is important economically because it diminishes the capability of individuals who are affected to perform physical labor, and it diminishes both growth and learning in children.
Posthemorrhagic anemia is discussed in this article because it is an important cause of iron deficiency. The acute and potentially catastrophic problems of hypoxia and shock that can occur from significant hemorrhage or severe iron deficiency are discussed elsewhere; however, daily blood losses can be small and may be overlooked.
Other groups at elevated risk for iron deficiency anemia include the following:
Adolescent girls with heavy menstrual bleeding
Patients with congestive heart failure
Renal transplant recipients
Elite runners and triathletes
Occasionally, patients with severe iron deficiency anemia from slow but persistent gastrointestinal (GI) bleeding have repeatedly negative testing of stool for hemoglobin. Therefore, it is important for the clinician to be aware of characteristics of the anemia at all intervals after the onset of bleeding.
Pathophysiology
Iron is vital for all living organisms because it is essential for multiple metabolic processes, including oxygen transport, DNA synthesis, and electron transport. Iron equilibrium in the body is regulated carefully to ensure that sufficient iron is absorbed in order to compensate for body losses of iron (see the image below). Whereas body loss of iron quantitatively is as important as absorption in terms of maintaining iron equilibrium, it is a more passive process than absorption.
The total body iron in a 70-kg man is about 4 g. This is maintained by a balance between absorption and body losses. Although the body only absorbs 1 mg daily to maintain equilibrium, the internal requirement for iron is greater (20-25 mg). An erythrocyte has a lifespan of 120 days so that 0.8% of red blood cells are destroyed and replaced each day. A man with 5 L of blood volume has 2.5 g of iron incorporated into the hemoglobin, with a daily turnover of 20 mg for hemoglobin synthesis and degradation and another 5 mg for other requirements. Most of this iron passes through the plasma for reutilization. Iron in excess of these requirements is deposited in body stores as ferritin or hemosiderin.
In healthy people, the body concentration of iron (approximately 60 parts per million [ppm]) is regulated carefully by absorptive cells in the proximal small intestine, which alter iron absorption to match body losses of iron (see the image below). Persistent errors in iron balance lead to either iron deficiency anemia or hemosiderosis. Both are disorders with potential adverse consequences.
Mucosal cells in the proximal small intestine mediate iron absorption. Intestinal cells are born in the crypts of Lieberkuhn and migrate to the tips of the villi. The cells are sloughed into the intestinal lumen at the end of their 2- to 3-day lifespan. Absorptive cells remain attuned to the body requirement for iron by incorporating proportionate quantities of body iron into the absorptive cells. This iron and recently absorbed iron decrease uptake of iron from the gut lumen by satiation of iron-binding proteins with iron, by stimulating an iron regulatory element, or both. The incorporation of iron into these cells in quantities proportional to body stores of iron also provides a limited method of increasing iron excretion in individuals replete in iron.
Either diminished absorbable dietary iron or excessive loss of body iron can cause iron deficiency. Diminished absorption usually is due to an insufficient intake of dietary iron in an absorbable form. Hemorrhage is the most common cause of excessive loss of body iron, but it can occur with hemoglobinuria from intravascular hemolysis. Malabsorption of iron is relatively uncommon in the absence of small bowel disease (sprue, celiac disease, regional enteritis) or previous GI surgery.
Iron uptake in the proximal small bowel occurs by 3 separate pathways (see the image below). These are the heme pathway and 2 distinct pathways for ferric and ferrous iron.
Three pathways exist in enterocytes for uptake of food iron. In the United States and Europe, most absorbed iron is derived from heme. Heme is digested enzymatically free of globin and enters the enterocyte as a metalloporphyrin. Within the cell iron is released from heme by heme oxygenase to pass into the body as inorganic iron. Most dietary inorganic iron is ferric iron. This can enter the absorptive cell via the integrin-mobilferrin pathway (IMP).Some dietary iron is reduced in the gut lumen and enters the absorptive cell via the divalent metal transporter-1 (DMT-1/DCT-1/Nramp-2). The proteins of both pathways interact within the enterocyte with paraferritin, a large protein complex capable of ferrireduction. Excess iron is stored as ferritin to protect the cell from oxidative damage. Iron leaves the cell to enter plasma facilitated by ferroportin and hephaestin, which associate with an apotransferrin receptor. The enterocyte is informed of body requirements for iron by transporting iron from plasma into the cell using a holotransferrin receptor.
In North America and Europe, one third of dietary iron is heme iron, but two thirds of body iron is derived from dietary myoglobin and hemoglobin. Heme iron is not chelated and precipitated by numerous dietary constituent that render nonheme iron nonabsorbable (see the image below), such as phytates, phosphates, tannates, oxalates, and carbonates. Heme is maintained soluble and available for absorption by globin degradation products produced by pancreatic enzymes. Heme iron and nonheme iron are absorbed into the enterocyte noncompetitively.
Dietary iron contains both heme and nonheme iron. Both chemical forms are absorbed noncompetitively into duodenal and jejunal mucosal cells. Many of the factors that alter the absorption of nonheme iron have little effect upon the absorption of heme iron because of the differences in their chemical structures. Iron is released from heme within the intestinal absorptive cell by heme oxygenase and then transferred into the body as nonheme iron. Factors affecting various stages of iron absorption are shown in this diagram. The simplest model of iron absorption must consider intraluminal, mucosal, and corporeal factors.
Heme enters the cell as an intact metalloporphyrin, presumably by a vesicular mechanism. It is degraded within the enterocyte by heme oxygenase with release of iron so that it traverses the basolateral cell membrane in competition with nonheme iron to bind transferrin in the plasma.
Ferric iron utilizes a different pathway to enter cells than ferrous iron. This was shown by competitive inhibition studies, the use of blocking antibodies against divalent metal transporter-1 (DMT-1) and beta3-integrin, and transfection experiments using DMT-1 DNA. This research indicated that ferric iron utilizes beta3-integrin and mobilferrin, while ferrous iron uses DMT-1 to enter cells.
Which pathway transports most nonheme iron in humans is not known. Most nonheme dietary iron is ferric iron. Iron absorption in mice and rats may involve more ferrous iron because they excrete moderate quantities of ascorbate in intestinal secretions. Humans, however, are a scorbutic species and are unable to synthesize ascorbate to reduce ferric iron.
Other proteins appear to be related to iron absorption. These are stimulators of iron transport (SFT), which are reported to increase the absorption of both ferric and ferrous iron, and hephaestin, which is postulated to be important in the transfer of iron from enterocytes into the plasma. The relationships and interactions among the newly described proteins are not known at this time and are being explored in a number of laboratories. [5]
The iron concentration within enterocytes varies directly with the body’s requirement for iron. Absorptive cells of iron-deficient humans and animals contain little stainable iron, whereas those of subjects who are replete in iron contain significantly higher amounts. Untreated phenotypic hemochromatosis creates little stainable iron in the enterocyte, similar to iron deficiency. Iron within the enterocyte may operate by up-regulation of a receptor, saturation of an iron-binding protein, or both.
In contrast to findings in iron deficiency, enhanced erythropoiesis, or hypoxia, endotoxin rapidly diminishes iron absorption without altering enterocyte iron concentration. This suggests that endotoxin and, perhaps, cytokines alter iron absorption by a different mechanism. This is the effect of hepcidin and the balance of hepcidin versus erythropoietin.
Most iron delivered to nonintestinal cells is bound to transferrin. Transferrin iron is delivered into nonintestinal cells via 2 pathways: the classical transferrin receptor pathway (high affinity, low capacity) and the pathway independent of the transferrin receptor (low affinity, high capacity). Otherwise, the nonsaturability of transferrin binding to cells cannot be explained.
In the classical transferrin pathway, the transferrin iron complex enters the cell within an endosome. Acidification of the endosome releases the iron from transferrin so that it can enter the cell. The apotransferrin is delivered by the endosome to the plasma for reutilization. The method by which the transferrin receptor–independent pathway delivers iron to the cell is not known.
Nonintestinal cells also possess the mobilferrin integrin and DMT-1 pathways. Their function in the absence of an iron-saturated transferrin is uncertain; however, their presence in nonintestinal cells suggests that they may participate in intracellular functions in addition to their capability to facilitate cellular uptake of iron.
Etiology
Dietary factors
Meat provides a source of heme iron, which is less affected by the dietary constituents that markedly diminish bioavailability than nonheme iron is. The prevalence of iron deficiency anemia is low in geographic areas where meat is an important constituent of the diet. In areas where meat is sparse, iron deficiency is commonplace.
Substances that diminish the absorption of ferrous and ferric iron include phytates, oxalates, phosphates, carbonates, and tannates (see the image below). These substances have little effect upon the absorption of heme iron. Similarly, ascorbic acid increases the absorption of ferric and ferrous iron and has little effect upon the absorption of heme iron.
Both nonheme iron and heme iron have 6 coordinating bonds; however, 4 of the bonds in heme bind pyrroles, making them unavailable for chelation by other compounds. Therefore, ascorbic acid chelates nonheme iron to enhance absorption but has no effect upon heme iron. Many dietary components, such as phytates, phosphates, oxalates, and tannates, bind nonheme iron to decrease nonheme iron absorption. They do not affect heme. This explains why heme is so effectively absorbed with foods containing these chelators. Iron hemoglobin structure.
Purified heme is absorbed poorly because heme polymerizes into macromolecules. Globin degradation products diminish heme polymerization, making it more available for absorption. They also increase the absorption of nonheme iron because the peptides from degraded globin bind the iron to prevent both precipitation and polymerization; thus, absorption of the iron in spinach is increased when the spinach eaten with meat. Heme and nonheme iron uptake by intestinal absorptive cells is noncompetitive.
Hemorrhage
Bleeding for any reason produces iron depletion. If sufficient blood loss occurs, iron deficiency anemia ensues (see the image below). A single sudden loss of blood produces a posthemorrhagic anemia that is normocytic. The bone marrow is stimulated to increase production of hemoglobin, thereby depleting iron in body stores. Once they are depleted, hemoglobin synthesis is impaired and microcytic hypochromic erythrocytes are produced.
Sequential changes in laboratory values following blood loss are depicted. A healthy human was bled 5 L in 500-mL increments over 45 days. A moderate anemia ensued, initially with normal cellular indices and serum iron. Subsequently, the mean corpuscular volume (MCV) increased as iron was mobilized from body stores and reticulocytosis occurred. The serum iron decreased, followed by an increase in the total iron-binding capacity. Gradual decreases in the red blood cell indices occurred, with maximal microcytosis and hypochromia present 120 days after bleeding. Values returned to normal approximately 250 days after blood loss. At the end of the experiment, iron was absent from body stores (marrow) because hemoglobin has a first priority for iron. Iron-59 absorption was increased after all values returned to normal in order to replenish the body store with iron. This suggests that the serum iron, total iron-binding capacity, hemoglobin concentration, and indices were not the primary regulators of iron absorption.
Maximal changes in the red blood cell (RBC) cellular indices occur in approximately 120 days, at a time when all normal erythrocytes produced prior to the hemorrhage are replaced by microcytes. Before this time, the peripheral smear shows a dimorphic population of erythrocytes, normocytic cells produced before bleeding, and microcytic cells produced after bleeding. This is reflected in the red blood cell distribution width (RDW); thus, the earliest evidence of the development of an iron-deficient erythropoiesis is seen in the peripheral smear, in the form of increased RDW.
Hemosiderinuria, hemoglobinuria, and pulmonary hemosiderosis
Iron deficiency anemia can occur from loss of body iron in the urine. If a freshly obtained urine specimen appears bloody but contains no red blood cells, suspect hemoglobinuria. Obtain confirmation in the laboratory that the pigment is hemoglobin and not myoglobin. This can be accomplished easily because 60% ammonium sulfate precipitates hemoglobin but not myoglobin.
Hemoglobinuria classically is ascribed to paroxysmal nocturnal hemoglobinuria, but it can occur with any brisk intravascular hemolytic anemia. In the early days of heart surgery with implantation of artificial valves, this mechanism of producing iron deficiency anemia was commonplace in large university hospitals. Today, with better prostheses, it has become a less frequent clinical problem. With less severe hemolytic disorders, there may be no significant hemoglobinuria.
Investigate renal loss of iron by staining the urine sediment for iron. Hemosiderin is detected intracellularly. Most of these patients have a low or absent plasma haptoglobin. Similarly, pulmonary hemosiderosis can result in sufficient loss of iron as hemosiderin from the lungs.
Malabsorption of iron
Prolonged achlorhydria may produce iron deficiency because acidic conditions are required to release ferric iron from food. Then, it can be chelated with mucins and other substances (eg, amino acids, sugars, amino acids, or amides) to keep it soluble and available for absorption in the more alkaline duodenum.
Starch and clay eating produce malabsorption of iron and iron deficiency anemia. Specific inquiry is required to elicit a history of either starch or clay eating because patients do not volunteer the information.
Extensive surgical removal of the proximal small bowel or chronic diseases (eg, untreated sprue or celiac syndrome) can diminish iron absorption. Rarely, patients with no history of malabsorption have iron deficiency anemia and fail to respond to oral iron therapy. Most merely are noncompliant with therapy.
Before placing these patients on parenteral therapy, document iron malabsorption either by measuring absorption of radioiron or by obtaining a baseline fasting serum-iron concentration and repeating the test 30 minutes and 1 hour after administration of a freshly prepared oral solution of ferrous sulfate (50-60 mg of iron) under observation. The serum iron should increase by 50% over the fasting specimen.
Iron-refractory iron deficiency
Iron-refractory iron deficiency anemia (IRIDA) is a hereditary disorder marked by with iron deficiency anemia that is typically unresponsive to oral iron supplementation and may be only partially responsive to parenteral iron therapy. IRIDA results from variants in the TMPRSS6 gene that lead to uninhibited production of hepcidin. IRIDA is characterized by microcytic, hypochromic anemia and serum hepcidin values that are inappropriately high for body iron levels.
Most patients with IRIDA are women. Age at presentation, disease severity, and response to iron supplementation are highly variable, even within families, with a few patients responding to oral iron but most requiring parenteral iron supplementation. [6]
An uncommon form of IRIDA occurs in postmenopausal women with androgen deficiency that leads to primary defective iron reutilization. This condition only responds to androgen replacement. [7, 8]
Epidemiology
United States statistics
In North America and Europe, iron deficiency is most common in women of childbearing age and as a manifestation of hemorrhage. Iron deficiency caused solely by diet is uncommon in adults in countries where meat is an important part of the diet. Depending upon the criteria used for the diagnosis of iron deficiency, approximately 4-8% of premenopausal women are iron deficient. In men and postmenopausal women, iron deficiency is uncommon in the absence of bleeding.
International statistics
A study of national primary care database for Italy, Belgium, Germany, and Spain determined that annual incidence rates of iron deficiency anemiaI ranged from 7.2 to 13.96 per 1,000 person-years. Higher rates were found in females, younger and older persons, patients with gastrointestinal diseases, pregnant women and women with a history of menometrorrhagia, and users of aspirin and/or antacids. [9]
In countries where little meat is in the diet, iron deficiency anemia is 6-8 times more prevalent than in North America and Europe. This occurs despite consumption of a diet that contains an equivalent amount of total dietary iron; the reason is that heme iron is absorbed better from the diet than nonheme iron. In studies of children and adolescents from Sudan and Nepal, iron deficiency anemia was found in as many as two thirds of subjects. [10]
In certain geographic areas, intestinal parasites, particularly hookworm, worsen the iron deficiency because of blood loss from the GI tract. Anemia is more profound among children and premenopausal women in these environs.
Age-related demographics
Healthy newborn infants have a total body iron of 250 mg (80 ppm), which is obtained from maternal sources. This decreases to approximately 60 ppm in the first 6 months of life, while the baby consumes an iron-deficient milk diet. Infants consuming cow milk have a greater incidence of iron deficiency because bovine milk has a higher concentration of calcium, which competes with iron for absorption. Subsequently, growing children must obtain approximately 0.5 mg more iron daily than is lost in order to maintain a normal body concentration of 60 ppm.
During adult life, equilibrium between body loss and gain is maintained. Children are more likely to develop iron deficiency anemia. In certain geographic areas, hookworm adds to the problem. Children are more likely to walk in soil without shoes and develop heavy infestations.
During childbearing years, women have a high incidence of iron deficiency anemia because of iron losses sustained with pregnancies and menses.
Gastrointestinal neoplasms become increasingly more prevalent with each decade of life. They frequently present with GI bleeding that may remain occult for long intervals before it is detected. Usually, bleeding from neoplasms in other organs is not occult, prompting the patient to seek medical attention before developing severe iron depletion. Investigate the etiology of the iron deficiency anemia to evaluate for a neoplasm.
Sex-related demographics
An adult male absorbs and loses about 1 mg of iron from a diet containing 10-20 mg daily. During childbearing years, an adult female loses an average of 2 mg of iron daily and must absorb a similar quantity of iron in order to maintain equilibrium. Because the average woman eats less than the average man does, she must be more than twice as efficient in absorbing dietary iron in order to maintain equilibrium and avoid developing iron deficiency anemia.
Healthy males lose body iron in sloughed epithelium, in secretions from the skin and gut lining, and from small daily losses of blood from the GI tract (0.7 mL daily). Cumulatively, this amounts to 1 mg of iron. Males with severe siderosis from blood transfusions can lose a maximum of 4 mg daily via these routes without additional blood loss.
A woman loses about 500 mg of iron with each pregnancy. Menstrual losses are highly variable, ranging from 10 to 250 mL (4-100 mg of iron) per period. These iron losses in women double their need to absorb iron in comparison to males. A special effort should be made to identify and treat iron deficiency during pregnancy and early childhood because of the effects of severe iron deficiency upon learning capability, growth, and development.
Race-related demographics
Race probably has no significant effect upon the occurrence of iron deficiency anemia; however, because diet and socioeconomic factors play a role in the prevalence of iron deficiency, it more frequently is observed in people of various racial backgrounds living in poorer areas of the world.
Prognosis
Iron deficiency anemia is an easily treated disorder with an excellent outcome; however, it may be caused by an underlying condition with a poor prognosis, such as neoplasia. Similarly, the prognosis may be altered by a comorbid condition such as coronary artery disease. Promptly and adequately treat a patient with iron deficiency anemia who is symptomatic with such comorbid conditions.
Chronic iron deficiency anemia is seldom a direct cause of death; however, moderate or severe iron deficiency anemia can produce sufficient hypoxia to aggravate underlying pulmonary and cardiovascular disorders. Hypoxic deaths have been observed in patients who refuse blood transfusions for religious reasons. Obviously, with brisk hemorrhage, patients may die from hypoxia related to posthemorrhagic anemia.
Whereas a number of symptoms, such as ice chewing and leg cramps, occur with iron deficiency, the major debility of moderately severe iron deficiency is fatigue and muscular dysfunction that impairs muscular work performance.
In children, the growth rate may be slowed, and a decreased capability to learn is reported. In young children, severe iron deficiency anemia is associated with a lower intelligence quotient (IQ), a diminished capability to learn, and a suboptimal growth rate.
Patient Education
Physician education is needed to ensure a greater awareness of iron deficiency and the testing needed to establish the diagnosis properly. Physician education also is needed to investigate the etiology of the iron deficiency.
Public health officials in geographic regions where iron deficiency is prevalent need to be aware of the significance of iron deficiency, its effect upon work performance, and the importance of providing iron during pregnancy and childhood. The addition of iron to basic foodstuffs is employed in these areas to diminish the problem.